Equipment Used in SuChAQuality
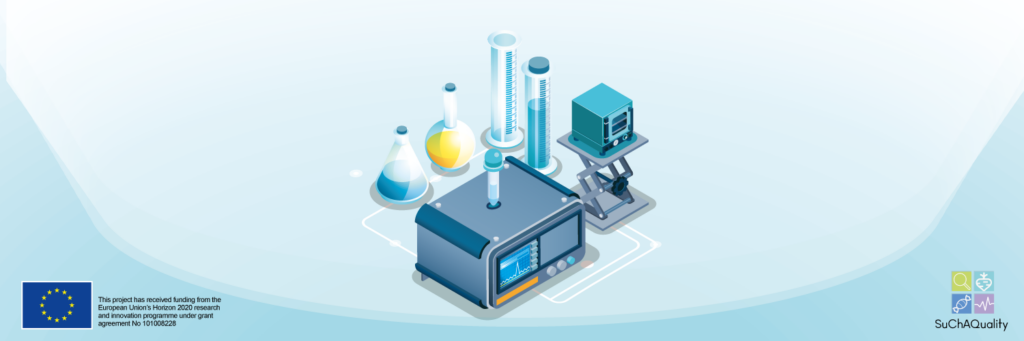
In the food industry and food science, different instruments can be used to make quantitative, qualitative analyzes of foods. The method and equipment to be used can be chosen according to various parameters such as reliability, required time, effectiveness, and cost. These devices, which we can perform molecular analysis, have different working principles. The devices we will examine in the following are IR-MS, Time Domain NMR, FFC-NMR, FTIR, NIR Spectroscopy, X-ray Diffraction, MRI, respectively.
Mass spectrometry is applied to qualify materials, identify unknown compounds in food samples, and explicate different structure of molecules and chemical characteristics (Milman, 2015). The sample is converted into gaseous ions, with or without fragmentation, to be characterized by their mass-to-charge ratio (m/z) (Muccio & Jackson, 2009). The conversion depends on the chemical compositions of the samples through interaction with the electron beam in the ion source. The working principle of mass spectrometry is based on the chemical reactions in the gas phase in which molecules of samples form ionic and natural species. On the other hand, a wide range of materials has isotopic variations which are unique to the root of the substance. This broad isotopic profile gives isotope ratio mass spectrometry (IRMS) a far-going application. IRMS provides a precise measurement for the minor differences between the isotopes such as 2H/1H, 13C/12C, and 18O/16O (Muccio & Jackson, 2009). The ions of a gaseous sample are focused through lenses and accelerated by supplying high voltage (Kaklamanos et al., 2020). The ions move through a magnetic field before being detected by the Faraday cups. The trajectory of the ions and which ions will reach the detector is determined by the accelerating voltage and the magnetic field strength. Multiple collectors are utilized to obtain simultaneous measurements of the intensity of several ion ratios. Each cup pair with its amplifier with a variant gain, and the IMRS data system collects and records the signals to produce a chromatogram for ions of a given mass-to-charge ratio m/z (Kaklamanos et al., 2020).
Time-domain NMR is also known as low field NMR since TD-NMR measurements are widely applied for low field NMR studies. The basic principle of time-domain NMR is the molecular mobility distinction between different components of food (Tang et al., 2019). This distinction is known as the relaxation time of T1 (spin-lattice) and T2 (spin-spin) and is measured through different pulse sequences. Time-domain NMR is an advantageous technique to measure bulk components such as water, carbohydrate, and fat protons in foods (Engelsen & van den Berg, 2017). In order to establish detailed quantitative structural and compositional information produced by TD-NMR experiments, adequate data-analysis techniques are required. Time-domain NMR experiments are relatively cost-friendly and provide a quantitative analysis of foods with easier operations. Moreover, TD-NMR methods offer rapid results and do not need any preliminary sample preparation (Berk et al., 2021).
Field-cycling NMR relaxometry is a technique that measures longitudinal T1 relaxation time over a wide frequency range using a unique instrument (Steele & Ferrante2, 2018). For the fast field-cycling (FFC) technique, the magnet must be capable of the fast electrical switching of B0 field (Galuppini et al., 2021). Fast field-cycling NMR relaxometry is the unique technique that allows the nuclear spin relaxation times measurement over a wide variety of magnetic field strength with only one instrument (Steele et al., 2015). Therefore, it provides a more plenitude investigation of molecular dynamics for the various substances. This technique requires a stable and precise acquisition. Even though FFC found a ground in food science for the characterization and quality measurements, it is not common. Possible applications are identification and distinction between different molecular dynamics, e.g., fast/slow dynamics, inter-molecular/intra-molecular dynamics; characterization of translational diffusion in bulk and geometrically restricted environment; separation of amorphous crystalline contributions in solids. All these features make FFC a valuable technique for the confectionery industry.
Fourier Transform Infrared (FTIR) spectroscopy is a physicochemical method based on the principle of measuring the vibration of chemical bonds with the absorption of infrared (IR) radiation (Basyigit Kilic & Karahan2, 2010). Infrared radiation is absorbed by different vibrational movements of chemical bonds such as stretching, shrinking, and bending. Spectral peaks occur in the infrared region with this vibration of chemical bonds and absorption properties. Used for quantitative quality control measurements in the food industry, the FTIR instrument combined with attenuated total reflection (ATR) technology significantly simplifies sample handling (van de Voort, 1992). For commercial gelatin, which is frequently used in the confectionery industry, these analyzes can be easily performed with the FTIR instrument (Rahman & Guizani, 2012).
Near-Infrared Spectroscopy (NIR) is another rapid quality measurement instrument that substantially reduces the measurement duration. Different products absorb near-infrared radiation at a specific wavelength (Patel, 2017). The NIR region mainly consists of overtones and combination bands due to hydrogen vibrations, and C-H, O-H, N-H bonds are strongly absorbed by NIR radiation (REICH, 2005). The absorbance composition of all molecular bonds in the sample forms the NIR spectrum of the sample. In the confectionary sector, rheological analysis of chocolate can be done with NIR spectroscopy, such as sucrose value in chocolate mass. Moreover, beet and cane sugar determination can be studied with this spectroscopy.
The X-ray system is frequently applied in quality and safety evaluation in food science, such as screening of foreign bodies and microparticles and density measurements by way of their imaging, diffraction, or scattering modes (Purohit et al., 2019). With the advanced technology of X-ray systems, qualitative analysis can be applied without destroying food. X-ray diffraction is a feature of different X-ray applications. X-ray diffraction is mainly used to understand crystalline polymorphism, crystalline fraction, and amorphous content. The area under the crystalline peak and total diffractogram and peak intensity are used to determine the crystallinity of food material (Miao & Roos, 2005). The principle of X-ray diffraction is to analyze the diffracted intensity of an X-ray beam that hits a sample that changes with the varying incident and scattered angle, polarization, and wavelength (Purohit et al., 2019).
Magnetic Resonance Imaging is fundementally developed for the medical studies, however, it was started to use a wide variety of diciplines over the time. MRI gives an opportunity to analyze foods and image the dynamic interactions in which occur as food processed or stored without any destruction or invasion. MRI based on the similar working principle with NMR (Schmidt et al., 1996). MRI is to provide two- or three-dimensional images of the material whereas NMR aims to determine chemical structure of the food. In MRI system, the atomic particles and an external magnetic field interact with each other. An energy is emitted by particles at particular frequencies, and intesity of emitted signal reflects the imaged structure (Ebrahimnejad et al., 2018).
Various quantitative and qualitative measurements are done for quality control applications, a critical part of the food industry. It is essential to make these measurements correctly with the proper device to understand food better and minimize possible risks.
REFERENCES
Basyigit Kilic, G., & Karahan2, A. G. (2010). Review of Fourier Dönüşümlü Kizilötesi̇ (Ftir) Spektroskopi̇si̇ Ve Lakti̇k Asi̇t Bakteri̇leri̇ni̇n Tanisinda Kullanilmasi. Dergi Par, 35(6).
Berk, B., Grunin, L., & Oztop, M. H. (2021). A non-conventional TD-NMR approach to monitor honey crystallization and melting. Journal of Food Engineering, 292, 110292. https://doi.org/10.1016/j.jfoodeng.2020.110292
Ebrahimnejad, H., Ebrahimnejad, H., Salajegheh, A., & Barghi, H. (2018). Use of Magnetic Resonance Imaging in Food Quality Control: A Review. Journal of biomedical physics & engineering, 8(1), 127–132.
Engelsen, S. B., & van den Berg, F. W. (2017). Quantitative analysis of time domain NMR relaxation data. Modern Magnetic Resonance, 1–19. https://doi.org/10.1007/978-3-319-28275-6_21-1
Galuppini, G., Magni, L., & Ferrante, G. (2021). The field-frequency lock for fast field cycling magnetic resonance: From NMR to MRI. Frontiers in Physics, 9. https://doi.org/10.3389/fphy.2021.688479
Kaklamanos, G., Aprea, E., & Theodoridis, G. (2020). Mass spectrometry: Principles and instrumentation. Chemical Analysis of Food, 525–552. https://doi.org/10.1016/b978-0-12-813266-1.00011-5
Miao, S., & Roos, Y. H. (2005). Crystallization kinetics and X-ray diffraction of crystals formed in amorphous lactose, Trehalose, and lactose/trehalose mixtures. Journal of Food Science, 70(5). https://doi.org/10.1111/j.1365-2621.2005.tb09976.x
Milman, B. L. (2015). General principles of identification by mass spectrometry. TrAC Trends in Analytical Chemistry, 69, 24–33. https://doi.org/10.1016/j.trac.2014.12.009
Muccio, Z., & Jackson, G. P. (2009). Isotope ratio mass spectrometry. The Analyst, 134(2), 213–222. https://doi.org/10.1039/b808232d
Patel, H. J. (2017). Near infrared spectroscopy: Basic principles and use in tablet evaluation. International Journal of Chemical and Life Sciences, 6(2), 2006. https://doi.org/10.21746/ijcls.2017.2.1
Purohit, S. R., Jayachandran, L. E., Raj, A. S., Nayak, D., & Rao, P. S. (2019). X-ray diffraction for Food Quality Evaluation. Evaluation Technologies for Food Quality, 579–594. https://doi.org/10.1016/b978-0-12-814217-2.00022-6
Rahman, M. S., & Guizani, N. (2012). Fourier transform infrared (FTIR) spectroscopic study of extracted gelatin from shaari (Lithrinus microdon) skin: Effects of extraction conditions. International Food Research Journal, 19(3)116-1173. Retrieved from http://www.ifrj.upm.edu.my/19%20(03)%202012/(51)%20IFRJ%2019%20(03)%202012%20Rahman%20Oman.pdf
REICH, G. (2005). Near-infrared spectroscopy and imaging: Basic principles and pharmaceutical applications. Advanced Drug Delivery Reviews, 57(8), 1109–1143. https://doi.org/10.1016/j.addr.2005.01.020
Rodrigues, C., Maia, R., Lauteri, M., Brugnoli, E., & Máguas, C. (2013). Stable isotope analysis. Food Protected Designation of Origin – Methodologies and Applications, 77–99. https://doi.org/10.1016/b978-0-444-59562-1.00004-9
Schmidt, S. J., Sun, X., Litchfield, J. B., & Eads, T. M. (1996). Applications of magnetic resonance imaging in food science. Critical Reviews in Food Science and Nutrition, 36(4), 357–385. https://doi.org/10.1080/10408399609527730
Steele, R., & Ferrante, G. (2018). Fast Field Cycling NMR Relaxometry – from molecular dynamics to practical applications.
Steele, R. M., Korb, J.-P., Ferrante, G., & Bubici, S. (2015). New applications and perspectives of Fast Field Cycling NMR relaxometry. Magnetic Resonance in Chemistry, 54(6), 502–509. https://doi.org/10.1002/mrc.4220
Tang, F., Vasas, M., Hatzakis, E., & Spyros, A. (2019). Magnetic resonance applications in food analysis. Annual Reports on NMR Spectroscopy, 239–306. https://doi.org/10.1016/bs.arnmr.2019.04.005
van Duynhoven, J., Voda, A., Witek, M., & Van As, H. (2010). Time-domain NMR applied to food products. Annual Reports on NMR Spectroscopy, 145–197. https://doi.org/10.1016/s0066-4103(10)69003-5
van de Voort, F. R. (1992). Fourier transform infrared spectroscopy applied to food analysis. Food Research International, 25(5), 397–403. https://doi.org/10.1016/0963-9969(92)90115-l
The information contained in this document reflects only the view of the SuChAQuality project and in no way reflects the European Commission’s opinion for which cannot be held responsible for any use that may be made of the information it contains